Kill the weeds, spare the grass
New tumor-targeting strategies limit damage to healthy tissues
June 2, 2016 | Leigh MacMillan
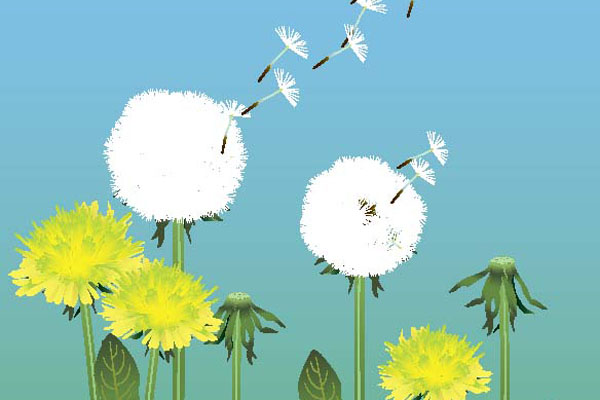
Illustration by iStock
When a pesky weed pops up in the midst of your lush lawn, what do you do? You probably don’t douse the yard with an herbicide that kills every green growing thing.
But that sort of indiscriminate damage is what cytotoxic chemotherapies do as they flow through the body. They kill dividing cells—both tumor cells and healthy cells, leaving a trail of destruction and side effects in their wake. These chemotherapies are the standard of care for many cancers simply because there are no other options.
This issue of Momentum highlights a few new strategies that aim—like selective weed-killers—to kill tumors while limiting the damage to healthy tissues.
Cancer-killing viruses
One new cancer drug is using a modified virus to kill cancer cells—a strategy that doctors first tried more than a century ago.
Back then, doctors attempted to harness the cancer-killing potential of viruses by using body fluids containing bacteria and viruses to transmit infections to cancer patients. Usually, the patient’s immune system killed the pathogens, but in some immunosuppressed patients, the infection persisted and tumors shrank. Side effects from the infection of normal tissues, however, were too severe for the therapy to be useful.
In recent decades, a molecular understanding of virus biology and the ability to alter viral genes renewed interest in the development of oncolytic—cancer-lysing—viruses for cancer therapy.
T-VEC is the first such virus to gain U.S. Food and Drug Administration approval for the treatment of melanoma in patients with inoperable tumors. It was approved in October 2015. T-VEC may also be useful for other cancers that are amenable to direct injection of the tumors.
“T-VEC is a unique drug; it’s a first-in-class oncolytic virus,” said Igor Puzanov, M.D., associate professor of Medicine at Vanderbilt. Puzanov began participating in clinical trials testing T-VEC in 2009.
T-VEC, short for talimogene laherparepvec, is a genetically modified herpes simplex virus type 1—the virus that causes cold sores. The modifications prevent the T-VEC virus from causing herpes, make it more selective for cancer cells, and introduce the gene for GM-CSF, a protein that stimulates an immune response.
T-VEC is injected directly into melanoma lesions, where it infects tumor cells and prompts production of GM-CSF. The virus ultimately causes the tumor cells to rupture (lyse), releasing bits and pieces of the cancer cells.
Dendritic cells of the immune system, which are attracted to the site by the GM-CSF, take up the dead cell bits and present them as “antigens” to killer T cells, which then recognize, attack and destroy tumor cells throughout the body.
“The beauty of this is that as the virus lyses the tumor cells, it is stimulating the patient’s immune system with the right set of antigens—not a set of antigens that we selected in a laboratory, but the real antigens from the real tumor,” Puzanov said.
In the Phase III clinical trial that paved the way for FDA approval of T-VEC, patients with melanoma lesions that could not be surgically removed received either T-VEC injected directly into their tumors or GM-CSF injected under the skin nearby. Patients receiving T-VEC were more likely to have an objective response of at least 30 percent tumor shrinkage with minimal side effects, and there was a trend toward extended overall survival.
T-VEC is currently being tested in combination with other “immunotherapy” drugs that spark the immune system to attack tumors. These include the checkpoint inhibitor drugs ipilimumab and pembrolizumab.
Douglas Johnson, M.D., assistant professor of Medicine, is leading the ongoing studies at Vanderbilt-Ingram Cancer Center.
The early data from these trials show promise for combination therapies including T-VEC, with more than half of patients responding, Puzanov said.
“I think the future is bright for oncolytic viruses such as T-VEC,” Puzanov said.
T-VEC requires specialized storage and injection protocols and is now being offered to patients as a standard of care through specialized centers like the Vanderbilt-Ingram Melanoma Program.
Delivering drugs directly to brain tumors
The blood-brain barrier—composed of tightly connected cells lining the blood vessels—is an important protection for the brain, shielding it from bacterial infections and biological toxins. But in keeping out large molecules, it prevents chemotherapy drugs from reaching tumors inside the brain.
“What we’re seeing now in diseases like lung cancer or breast cancer is that we’ve improved systemic chemotherapy options, but the brain remains a reservoir for cancer cells—whatever disease is there does not respond to that chemotherapy, and we see relapse in the brain,” said Lola Chambless, M.D., assistant professor of Neurological Surgery.
Primary brain tumors—glioblastoma and other gliomas—don’t have good treatment options either, Chambless said.
“Glioblastoma has a median survival of about 14 months with total standard of care treatment—surgical resection, radiation and chemotherapy,” Chambless said. “There’s a lot of room for improvement there.”
Intra-arterial chemotherapy—delivery of drugs directly to a tumor through a catheter—may improve treatment options for tumors in the brain. It requires a team of physicians including oncologists and neurosurgical oncologists to diagnose and biopsy—or remove—a tumor, and endovascular neurosurgeons to thread catheters from the groin to the arteries of the brain.
“The concept of intra-arterial therapy in the central nervous system (CNS) is to bypass the systemic circulation, break down the blood-brain barrier selectively and push higher doses of chemotherapy directly into the tumor,” Chambless said.
Advances in endovascular techniques—improved wires and catheters—have made intra-arterial chemotherapy possible, said Rohan Chitale, M.D., assistant professor of Neurological Surgery and Radiology and Radiological Sciences.
“We have more capability for putting catheters where we want them; we can bring micro-catheters into the brain in the blood vessels that feed the tumor,” he said.
Chitale and Matthew Fusco, M.D., assistant professor of Neurological Surgery and Radiology and Radiological Sciences, normally use endovascular techniques to treat vascular problems like aneurysms and arteriovenous malformations—abnormal connections between arteries and veins.
They also use the techniques prior to surgery to cut off the blood supply to particular brain tumors that are highly vascular and pose high risks for surgery.
“Operating on some brain tumors can result in two to three liters of blood loss, making the surgery unsafe,” Fusco said. “We can go in first and block off the blood vessels to minimize blood loss and improve the safety of the surgery.”
Blocking blood flow is well established, Fusco said. The question now is “how do you transition that to deliver a specific agent,” he said.
Intra-arterial chemotherapy is being used for retinoblastoma tumors in the eye and in some peripheral tumors. Results also look strong for CNS lymphoma—a blood cancer of the brain that is diffuse and not a candidate for surgical removal, Chambless said.
Next up could be cancers that have metastasized to the brain, and in the longer run gliomas, where identifying effective anti-cancer drugs need to happen first.
“Once we identify some better drug options, this delivery method may make more sense,” Chambless said.
Nanoparticle drug shuttles
Sometimes a promising drug candidate can’t get where it needs to go. It might not dissolve in water, so it can’t be put into standard saline solutions used for intravenous injections. Or it might get digested into nonfunctional bits in the stomach or liver before it reaches its destination. Or it may go to the wrong place in the body altogether.
Craig Duvall, Ph.D., assistant professor of Biomedical Engineering, and his colleagues in the Advanced Therapeutics Laboratory at Vanderbilt University are developing nanoparticle drug delivery systems to overcome these and other pharmacological barriers.
Nanoparticles are small—between 10 and 200 nanometers in size—about a thousand times smaller than the width of a human hair. Duvall’s team builds nanoparticles using chemical “building blocks” that react to form polymer molecules that can then be assembled into nanoparticles. The researchers vary the blocks to modify the characteristics of the resulting particles. In general, nanoparticles have a core that contains the therapeutic compounds—often it is hydrophobic (fats and oils are hydrophobic)—surrounded by a water-soluble coating that may also contain specific targeting molecules.
“By optimizing just the stability, the size and the surface chemistry of the nanoparticles, you can make particles that will circulate for a long time and preferentially accumulate in tumors because of the characteristics of tumor blood vessels,” Duvall said.
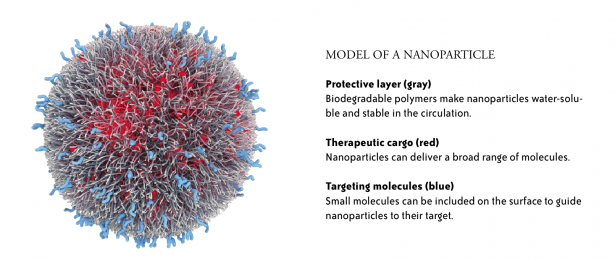
ACCURINS® nanoparticle image courtesy of BIND Therapeutics
In one set of studies, the researchers are incorporating gene-silencing molecules called small interfering RNAs (siRNAs) into the nanoparticles. Theoretically, siRNA molecules can be designed to silence any gene, but without a protective delivery system, they would be rapidly excreted by kidney filtration into the urine or simply destroyed in the circulation.
Duvall’s team is working with Rebecca Cook, Ph.D., and Dana Brantley-Sieders, Ph.D., Vanderbilt-Ingram breast cancer investigators, to target the molecule RICTOR, part of a signaling complex implicated in breast cancer called mTORC2.
Small molecule drugs that inhibit mTORC2 function have been developed, but they also block a related complex called mTORC1, and cause treatment-limiting side effects.
Using nanoparticles containing RICTOR-silencing siRNAs, the investigators have demonstrated anti-tumor effects in mouse models of breast cancer. Next, they will study the impact of the therapy on different types of breast cancer, and also in combination with standard chemotherapies.
Another series of studies, in collaboration with Julie Sterling, Ph.D., and Scott Guelcher, Ph.D., in the Vanderbilt Center for Bone Biology, is developing nanoparticles to deliver a drug candidate to breast cancer that has metastasized to bone.
Sterling and her colleagues identified inhibitors of the transcription factor GLI2, a protein that controls the expression of genes and is overexpressed in breast tumor cells in bone. Transcription factors and other proteins that operate inside cells are challenging targets, and the candidate GLI2 inhibitors are not soluble in water and cannot be effectively used in vivo. The inhibitors can’t make it past the physiology of the human body to reach these targets within cells.
Duvall and his team are incorporating GLI2 inhibitors into nanoparticles, and they are modifying the nanoparticle surface to bind more tightly to bone.
“We’re taking these new drugs that have been discovered, enabling their use in vivo just by solubilizing them, and then improving the targeting and retention of the drug at the tumor site in bone, so we’ll be able to get a better effect with a decreased dose and minimize non-specific side effects,” Duvall said.